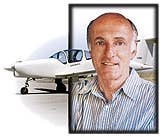
One reader castigated me for not paying enough attention to Thielert diesels. Another inquired whether the just-certified Diamond TwinStar would have superior climb performance because of the great torque of its Thielert diesel engines. A critical mass of Thielert interest has evidently been reached.
Thielert GmbH is located at the small town of Lichtenstein in the former East Germany (not to be confused with the principality of Liechtenstein, wedged almost invisibly between Switzerland and Austria). The company produces, among other things, liquid-cooled geared turbo-diesel aircraft engines based on Mercedes automotive designs. A 1.7-liter, 135-hp four-cylinder is currently certified for retrofit to Cessna 172s and Piper Warriors originally equipped with the 160-hp Lycoming O-320 engine. The Austrian firm of Diamond offers its single-engine Diamond Star with one of the engines, and the upcoming TwinStar has two. More than a hundred Thielert-powered airplanes are now flying, and the company expects to certify a 4.0-liter, 310-hp V-8 this year.
It's unusual to offer an engine of lower power to replace one of higher. One wonders where the company gets the chutzpah to do it. The answer is that, at least in the 172 and the Warrior, performance is barely affected, gaining a little in some areas and losing a little in others. To understand how this can be so when the new engine is apparently much weaker than the old, we need to look at the characteristics of diesel and gasoline engines.
To start with, their combustion cycles are fundamentally different. The gasoline engine draws a fuel-air mixture into the cylinder, compresses it, and finally ignites it with a spark. The need to avoid spontaneous ignition (either pre-ignition or detonation) limits the compression ratio, while the need to ignite and burn the compressed mixture requires that the ratio of fuel to air remain within fairly narrow limits. An air throttle, linked to a fuel valve in injected engines, regulates power, while a mixture control allows fine adjustment of fuel flow in order to ensure a combustible, but not wasteful, fuel-air ratio.
The Thielert engines have no air throttle. Only engine speed regulates the amount of air they take in, while fuel flow controls their power output. Rather than a fuel-air mixture, only air is compressed by the rising piston. A very high compression ratio-18:1, more than double that of a typical unsupercharged aircraft gasoline engine-raises the temperature of the air in the cylinder (because the same amount of heat energy gets crammed into a much smaller volume of air) above the point at which hydrocarbon fuels ignite spontaneously. No timed spark is needed; fuel, sprayed into the cylinder under extremely high pressure as the piston reaches the top of its travel, burns regardless of the fuel-air ratio, which is always, by gasoline-engine standards, very lean. There is no harm, after all, in having excess air being present, just so long as there is enough oxygen to react with all of the hydrocarbons in the fuel. Jet engines, too, take in far more air than they use for combustion.
In their familiar roles as powerplants for generators, trucks and ships, diesel engines are typically designed to be slow-turning but to have a long stroke. The stroke, which is the distance the piston travels, is twice the crankshaft's "throw"; the throw, in turn, is the maximum length of the lever arm against which the piston exerts its pressure. Long throws ensure that diesels yield plentiful torque. Since horsepower is the product of torque and engine speed, a slow-turning engine with lots of torque can work as hard (that is, produce the same horsepower) as a faster-turning engine with lower torque. Torque is naturally determined not by crank geometry alone, however, but also by the pressure in the cylinder during the power stroke and by the area of the piston (which is as much as to say the "bore" of the engine).
Although the 135-hp Thielert Centurion 1.7's weight, around 300 pounds, is similar to that of the 160-hp Lycoming O-320, its displacement is less than a third of the Lycoming's. It achieves its maximum power at 2300 prop rpm-3900 crank rpm-versus 2700 for the Lycoming.
While it is tempting to suppose that the key to the similarity in takeoff and climb performance between the Lycoming- and Thielert-powered versions of the 172 and the Warrior lies in the diesel's superior torque, in fact both engines deliver about the same torque-around 300 pound-feet-to the propeller. I suspect that the explanation is really more mundane. The Thielert engine uses an automatic variable-pitch propeller, whereas the Lycoming, in these airplanes, drives a fixed-pitch prop. During takeoff and climb, the Lycoming does not achieve maximum rpm, whereas the Thielert does. The superior aerodynamic efficiency of the Thielert's propeller nearly erases whatever residual power advantage the Lycoming might have, and so the takeoff distance and rates of climb for the two engine types turn out to be quite similar, with the Thielert lagging a bit at low altitude and outdistancing the Lycoming higher up. I suspect that a 172 or Warrior with a constant-speed prop would handily outclimb both its fixed-pitch counterpart and the Thielert-engined version at low altitude, because it would be able to make full use of its 160 horsepower at all speeds.
Propeller pitch corresponds to transmission gear ratio in a road vehicle. It's true that an engine with greater low-end torque, like a big truck's diesel, will be better able to keep up revolutions, and therefore power output, at low rotational speed. In road terms, we would say that it will not "lug" or "bog down" in the way a less torquey engine might if it were not provided with a sufficiently low gearing. A constant-speed prop is analogous to a continuously variable transmission, adjusting engine speed to provide the desired horsepower at all times. A car or truck with such a transmission, or with a sufficiently wide range of closely-spaced gears, would climb a hill rapidly even if it lacked low-end torque, because it would be able to keep its engine speed, and therefore its power output, high. It is not true that, given suitable transmissions, of two vehicles of equal horsepower the one with the higher torque will climb better. Climb is all about horsepower, not torque; in fact, the very definition of horsepower is based on the rate at which a weight can be raised.
With regard to fixed-pitch props, a "climb" prop is one that has sufficiently flat ("fine") pitch to allow the engine to develop high rpm at climbing speed. A "cruise" prop is coarsely pitched, to preclude its over-revving at cruising speed. Racing airplanes have props pitched for top speed, but they take off sluggishly; glider tugs have climb props and can't get up much steam for cruise. Normal passenger-carrying airplanes have compromise props that don't allow the engine to achieve full power during climb, and require that it be throttled back during cruise.
With regard to the TwinStar, one may wonder how a four-seat twin is going to achieve a positive climb rate on a single 135-hp engine. Presumably the folks at Diamond have worked out the answer. It can only be that superior aerodynamic efficiency, particularly in the form of a large wingspan, reduces the power required for climb. Merely to lift 3,000 pounds at, say, 250 fpm requires 23 horsepower, which translates into something closer to 30 when prop losses are taken into account. Airframe and cooling drag at 90 knots will probably require another 80 hp, which translates, again, into 100 or so from the engine.
The Thielert diesels come into their own in cruise. They have a genuine FADEC, or Full Authority Digital Engine Control-a single lever that electronically manages both fuel flow and rpm. Because the efficiency of an internal combustion engine improves as its compression ratio increases, diesels naturally burn less fuel per horsepower-hour than gasoline engines do. They also run on jet fuel, which currently happens to be 10 to 20 percent cheaper, on average, than avgas, or on road diesel fuel, which is cheaper still. Maximum power may be used at any time; the conventional limit of 75 percent for cruise does not apply. Being turbocharged, the diesel is essentially flat-rated over the range of altitudes that a 172 or Warrior would most often use, and so is faster than they are at high altitude. Throttled back to economy cruise, it achieves unusually long range-provided that the pilot has the Sitzfleisch to match.
It is not surprising that certified aviation diesels have appeared in Europe. The cost of fuel there is much higher than it is here, and so the prospect of significantly reduced direct operating costs softens the blow of the Thielert engines' price, which is higher than that of present gasoline-burning types. That they should be expensive is not surprising; they are complex, sophisticated machines in comparison with the rather simple ones they aim to replace.
Those Pesky V-speeds Several readers pounced on my statement that maneuvering speed drops with weight, pointing out that a just-short-of-stalling pull-up at the gross-weight maneuvering speed produces the same air loads on the wing regardless of the airplane weight, even though the occupants may experience a higher G force. True enough. But the wing spar is not the only structural component in the airplane. Many others are designed to support fixed loads. The engine mount, for example, is designed to support the engine at a certain load factor, and the higher acceleration experienced by a lighter airplane during a pull-up to a stall at the gross-weight maneuvering speed could exceed that factor.
One hawk-eyed observer, Dick Reilly, pointed out that my statement that all of the characteristic speeds of an airplane, including best rate and angle of climb speeds, are functions of angle of attack, does not hold true in practice for airplanes with fixed-pitch propellers. I would like to say that I had omitted to mention this caveat in the interest of keeping things clear, but actually I just never thought about it. Since the efficiency of the fixed-pitch prop depends on true rather than indicated airspeed, the indicated speeds for best rate and angle of climb are lower, at high altitude, than the appropriate angle of attack for those conditions would imply.
That may sound clear, but try and remember it the next time you're dragging a 172 over a saddle in building cu at 14,000 feet.


Sign-up for newsletters & special offers!
Get the latest FLYING stories & special offers delivered directly to your inbox