Why Jets Fly High and Fast
Peter Garrison explains how jets produce thrust and recips produce torque.
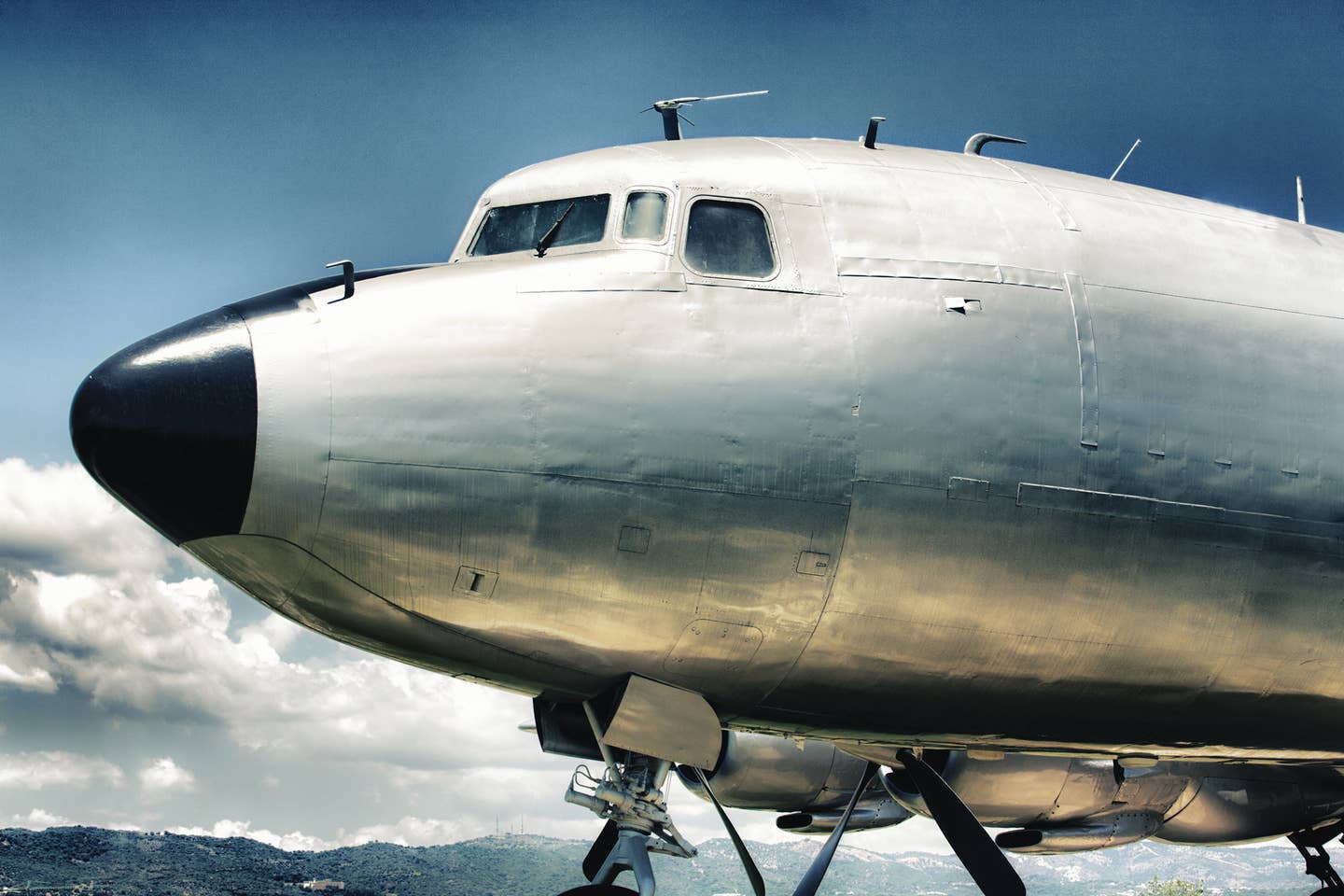
The bullet shaped body tows swirling air behind it. Hans Kandlbauer
We use the word “thrust” for the force that drives the airplane forward against air resistance. Because the word applies equally to all kinds of propulsion, comparisons of the properties of jets and props may include some statement to the effect that props produce their thrust by accelerating a mass of air backward.
This is true in the same sense that it is true that wings produce an upward force by accelerating air downward. But the thrust of a jet engine is different; it is produced directly, within the engine, and does not depend on an aerodynamic interaction with the surrounding air. In fact, the surrounding air is an impediment, in that it resists the outflow from the exhaust nozzle.
A jet engine creates thrust by taking in air, heating it so its pressure rises, and letting it spew out the back end at a higher velocity than that at which it came in. Fuel adds almost nothing to the mass passing through the engine; what is added is velocity, and this change in velocity produces an opposite reaction, as if you propelled a boat forward by tossing its cargo over the stern. (In case you ever wondered what in the engine this reaction is pushing against, it’s the compressor blades and the central structure that supports them.)
What a reciprocating engine—or a turbine driving a propeller—produces is not thrust but torque. It spins a shaft. To produce thrust, something must be added, namely, a propeller. Wings, helicopter rotors, propellers—they all do the same thing: exploit the pressure changes that occur when an inclined plane is made to move through a fluid.
Because a propeller depends on the surrounding fluid medium to produce thrust, whereas a jet does not, the two types of powerplant are differently affected by altitude.
The output of a jet engine declines, broadly speaking, in proportion to the density of the air in which it operates. You can’t turbocharge a jet engine because the engine itself is fundamentally a self-propelled turbocharger. On the other hand, at high speeds, a jet profits from ram effect, which its compressor compounds, while lower ambient density reduces resistance to the outflow from the exhaust nozzle. Jets therefore operate more efficiently—that is, require less fuel per unit of thrust—at high speed and altitude than they do when flying low and slow.
The situation of the propeller is different. The engine driving the propeller can be turbocharged and may maintain sea-level power up to a high altitude. But as the density of the air diminishes, the propeller blades have less to work with. Once they are at their optimal angle of attack, the only way to maintain thrust against increasingly rarefied air is to increase the area, number or speed of the propeller blades.
At the same time, as the airplane takes advantage of thinner air by increasing its true airspeed—which is the main reason for wanting to fly high in the first place—the pitch angle of the propeller blades, governed by both their own rotational velocity and the forward speed of the airplane, must increase. As blade pitch increases, the lift vector of each blade points farther and farther away from the thrust axis. This difficulty might be alleviated if it were actually possible to spin the propeller faster, but in fact, the faster the airplane goes, the slower the propeller must turn to keep its tip speeds away from the transonic drag rise. The efficiency of a given propeller therefore decreases as speed and altitude increase.
Read More from Peter Garrison: Technicalities
In short, it is just as you guessed: A jet engine gets happier with increasing altitude and speed, and a propeller engine gets sadder. A turbofan, a hybrid of the two, gets both at once—it’s a conflicted, bipolar thing, just like the rest of us.
A Postscript on Lift and Drag
In the March issue, I wrote about the word “lift” and how it improperly implies a force always acting upward. I solicited reader suggestions for alternative terms and received but one proposal: “suck,” which I do not think has much of a future. Actually, I’m not sure many readers care whether “lift” is a misnomer or not, so long as their wings keep lifting. One person who does care, however, is Terry Golden, from whom I got the idea of writing about the subject in the first place. Golden has campaigned tirelessly against the word “lift” and even hands out buttons proclaiming, “Lift Is False Terminology” (note the initials). Yet even he has not offered a convincing replacement.
In that article, I posed a question about drag. Suppose you took a streamlined body of revolution, such as a tip tank, and sliced it across at its thickest point. Which chunk would have more drag—the bullet-shaped front portion, with the smooth oval nose and the bluff aft end, or the one with the flat front and streamlined tail?
I thought the flat-nosed body would have less drag. But that turned out—after a series of computer simulations run by my friend Hans Kandlbauer—to be true only if you rounded the sharp edge of the front face. You don’t have to round it much; a radius of one-fiftieth of the body length was enough. A sharp edge produced tremendous drag, five times the drag of the bullet-shaped alternative. But with the rounded edge, the drag of the flat-faced body was a third less than that of the bullet. This finding hinted at why radial-engined fighters of World War II, with their flat fronts and rounded-edge cowlings, performed as well as they did, and also why it is desirable for trailers to have rounded front corners.
Drag is usually expressed as a coefficient—that is, a dimensionless number—by relating the measured drag to some area, called a “reference area.” For complete airplanes, the wing area is often the reference area, although in professional circles the total surface area of the airplane, or “wetted area,” is taken a little more seriously.
For streamlined bodies—such as fuselages or, say, an external radar pod—cross-sectional area is used; this is true in the automotive community as well. This approach makes sense because an external pod, a fuselage or an auto body is a streamlined fairing around some object or collection of objects with a known cross section.
For an external fuel tank, on the other hand, it would seem logical to relate the drag to the volume because that is what you want to get most of with the least penalty in drag.
It turns out that to envelop a certain volume, the original airfoil-shaped body of revolution is best. To provide a fairing around an object of a given cross section, however, a blunt or hemispherical nose and streamlined after body is slightly superior to the pure airfoil shape—probably because the predominant cause of drag is skin friction, and the blunter nose has somewhat less skin area for the air to rub against.
The lesson of all this is that low drag, at least at subsonic speeds, does not require long swoopy noses so much as gently tapering tails, as Charles Darwin evidently knew when he created the sperm whale.
This story appeared in the August 2021 issue of Flying Magazine


Subscribe to Our Newsletter
Get the latest FLYING stories delivered directly to your inbox