The Riddle of Takeoff Distance
Not all performance calculations are as specific as we think.
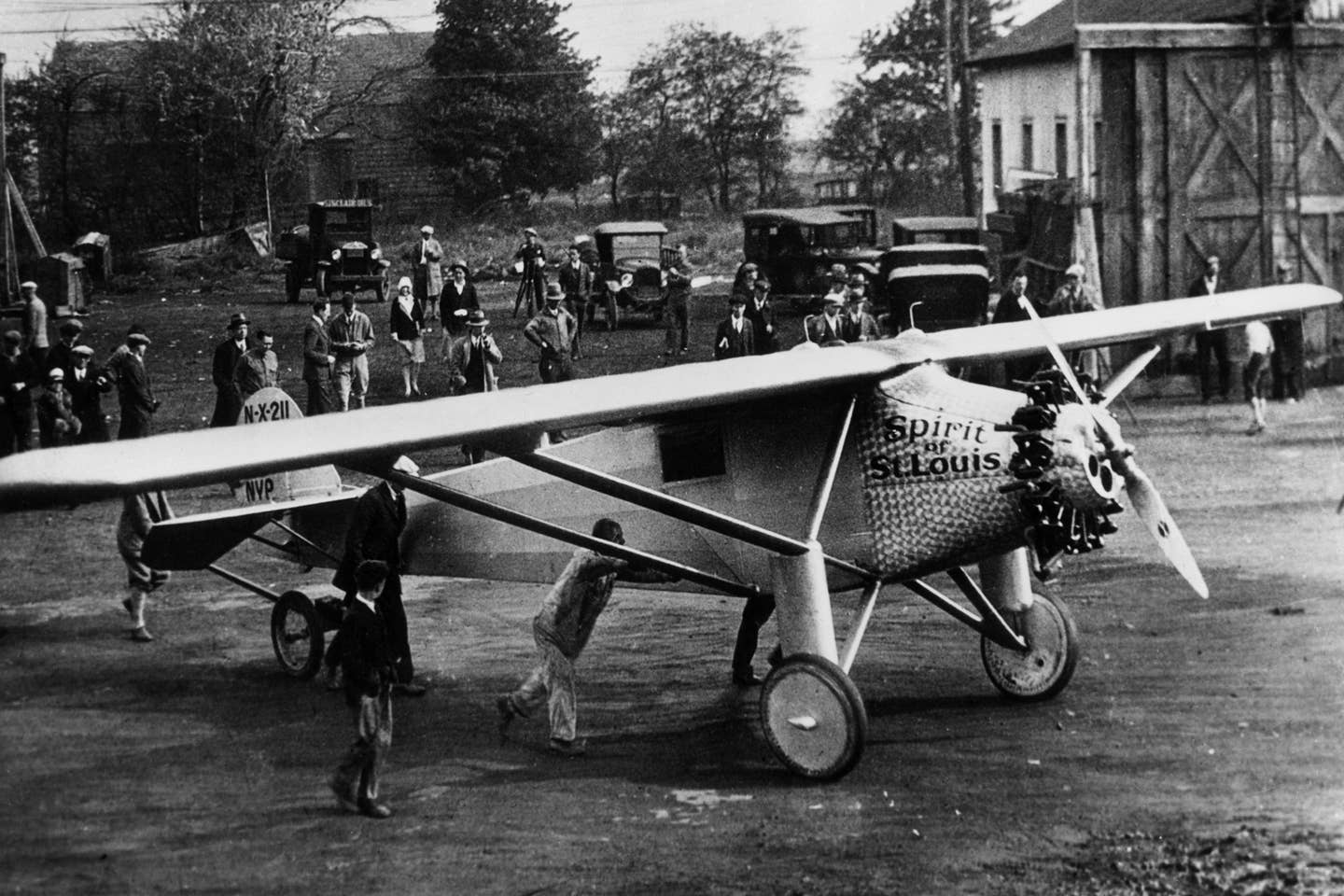
For the historic flight, takeoff distance was merely an educated guess. American Stock Archive
In Spring 1927, while Spirit of St. Louis was being built by Ryan Airline Company in San Diego, Charles Lindbergh corresponded with the Standard Steel Propeller Company of Pittsburgh regarding the best pitch setting for its ground-adjustable propeller blades.
The aluminum-alloy blades were off-the-shelf items, using a Clark Y airfoil and a twist distribution suitable for Ryan’s mail planes, upon which the design of Spirit was based. The Clark Y was a profile commonly used for propeller blades, not because it had any special aerodynamic advantage but because its flat bottom made fabricating blades and measuring their pitch angles easier.
Because the propeller’s pitch could not be changed in flight, Lindbergh had a ticklish decision to make. He could pitch the prop for best efficiency in cruise, but it would then be less suitable for takeoff; or he could pitch it for maximum takeoff thrust and give up some range. The airplane would weigh around 5,100 pounds and have a static thrust of around 700 pounds. It was not going to leap forward in any case. The runway at New York’s Roosevelt Field, where the flight would begin, was 5,000 feet long, with telephone lines just beyond the departure end. The takeoff for the Paris flight would be the airplane’s first at maximum weight. For it to not succeed would be fatal to not only the project but possibly the pilot as well.
The previous fall, the French World War I ace René Fonck, eyeing the same $25,000 Orteig prize as Lindbergh, had tried to take off from Roosevelt Field in a 28,000-pound trimotor Sikorsky sesquiplane. The effort failed spectacularly, with the airplane cartwheeling off the end of the runway and killing two of the four aboard. Not that Fonck’s debacle was because of the wrong propeller pitch setting—in fact, the landing gear failed under the overload of fuel. Nor, I suppose, did Lindbergh need reminding of the likely consequences of his own fuel-filled airplane failing to get off the ground.
The propeller company advised Lindbergh that a pitch setting of 15.5 degrees would be best for takeoff, but 16.5 degrees would give the best efficiency in cruise. Lindbergh opted for cruise.
Footage of the takeoff, readily found on YouTube, shows the airplane accelerating slowly at first, then gradually growing lighter on its wheels, bouncing several times, and—after what must have seemed to Lindbergh an eternity—embarking on a steady, shallow climb. It is impossible to judge the distance to the telephone wires, which are visible as Spirit passes over them. Wikipedia says the airplane became airborne 1,000 yards before the wires. Lindbergh himself, in his narrative of the flight, says 1,000 feet.
Apart from his later struggles with sleep, the takeoff is the most stressful part of Lindbergh’s account of his flight. Assuming that his description accurately reflects the event, he was uncertain that Spirit of St. Louis could get into the air. This may seem strange because he had devoted a great deal of time to calculating every aspect of his undertaking, but takeoff distance was impossible to calculate with any precision at all. The experience of painfully slow initial acceleration over muddy ground, with men pushing on the wing struts to help—and the impression of the hopeless inadequacy of the engine and propeller—were for a time extremely vexing.
The problem for Lindbergh was that takeoff distance is, as a classic textbook concedes, “perhaps the most difficult performance item to predict accurately.” The methods used may be mathematically rigorous, but analyses “are based on assumptions that are accurate only for special conditions of pilot technique, ground conditions, airplane attitude and drag, and average variations in effective thrust.” In other words, you may do a very fancy calculation and its result may not resemble the practical reality. Furthermore, intuitive notions of what’s important may be quite wrong.
Read More from Peter Garrison: Technicalities
In 1936, nine years after Lindbergh’s flight, a propeller specialist named Edwin Hartman at NACA’s Langley, Virginia, laboratory wrote Technical Note 557, a pithy 18-page study of the takeoff problem. Hartman disposed of several common misconceptions. One was that static thrust—this is the thing you get more of with a flatter propeller pitch—has a large effect on takeoff distance. It doesn’t so long as the cruising speed is not extremely far above the stalling speed, a condition more prevalent in 1936 than today. Spirit’s wasn’t. Furthermore, the effects of running up to full power before releasing the brakes, or beginning the takeoff from a rolling turn onto the runway, are also negligible. The reason is that very little distance is covered in the early part of the takeoff roll, so the initial acceleration is unimportant. What matters is the acceleration later, when the airplane is covering ground rapidly. That is when propeller efficiency, which depends largely on the blade pitch, counts and airframe drag plays an increasing role. The subjective impression of slow initial acceleration, which troubled Lindbergh, might be correct, but the assumption that it signals a long takeoff distance is not.
Hartman also dismissed the idea, current at the time, that the duration of the takeoff run of a seaplane was of fundamental importance. He speculated that this notion arose from a mistaken intuition that a longer time meant a longer distance. In fact, Hartman observed, a shorter takeoff time may actually correlate with a longer takeoff distance if more of the time is spent hurtling along at high speed but accelerating very little.
One of the variables by which the pilot affects the takeoff distance is the angle of attack at which the airplane is held during the roll. The lift coefficient for the minimum of rolling resistance plus induced drag is approximately 1.6 times the wing aspect ratio multiplied by the friction coefficient of the runway surface, which ranges from 0.02 for smooth pavement to 0.3 for mud or deep grass. This merely confirms the general sense that you hold the nose up for a soft-field takeoff. A corollary, however, is that on a smooth, hard surface, a taildragger ought to get its tail up as soon as possible; maintaining a three-point attitude in order to get lift earlier is a mistake.
Hartman provided an ingenious short method for predicting takeoff distance, but unfortunately, it requires knowing the rate of acceleration at 70 percent of liftoff speed, something that can be determined only with specialized instrumentation.
In 1936, it made sense to look for analytical solutions to the takeoff- distance problem—in other words, reducing it to an equation involving some known parameters. Today, we take a different approach. A digital computer—consulting tables of propeller efficiency against speed—computes thrust, drag, rolling resistance, and the effects of wind and runway incline at intervals of a tenth of a second, adding up distance traveled until arriving at the specified rotation speed.
I applied such a method to Spirit of St. Louis, making what I thought were reasonable assumptions about its parasite drag and propeller characteristics. I got a takeoff roll of 46 seconds and a distance of 2,600 feet.
If only Lindbergh had known, he would have been spared a bit of anxiety. He didn’t know, so he guessed—and, as usual with him, guessed well.
This story appeared in the September 2020 issue of Flying Magazine


Sign-up for newsletters & special offers!
Get the latest FLYING stories & special offers delivered directly to your inbox